Community Structure and Ecological and Behavioral
Traits of Ants (Hymenoptera: Formicidae) in Massachusetts Open and Forested Habitats
Israel Del Toro, Kevin Towle, Drew N. Morrison, and Shannon L. Pelini
Northeastern Naturalist, Volume 20, Issue 1 (2013): 103–114
Full-text pdf (Accessible only to subscribers.To subscribe click here.)

Access Journal Content
Open access browsing of table of contents and abstract pages. Full text pdfs available for download for subscribers.
Current Issue: Vol. 30 (3)
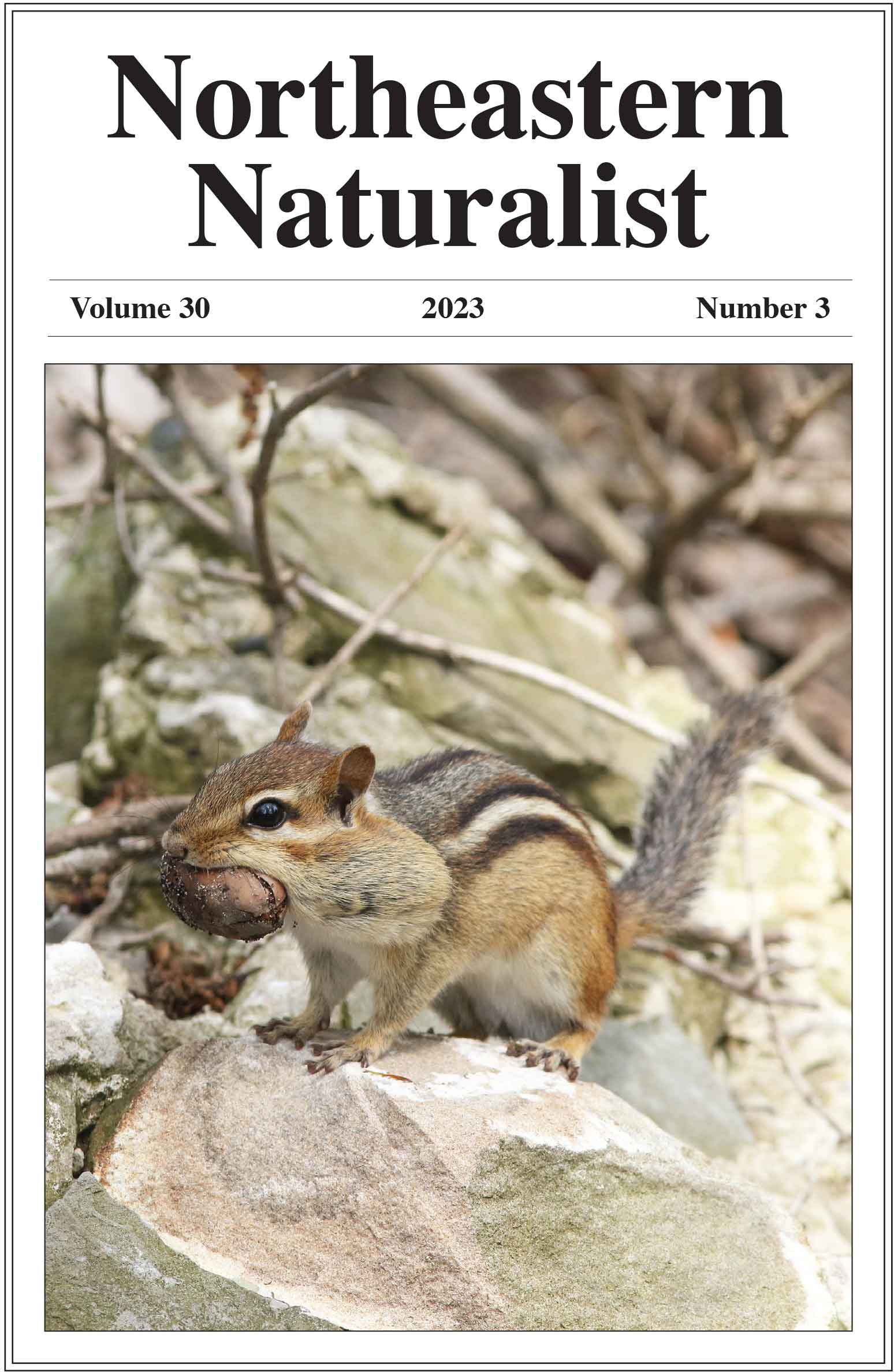
Check out NENA's latest Monograph:
Monograph 22
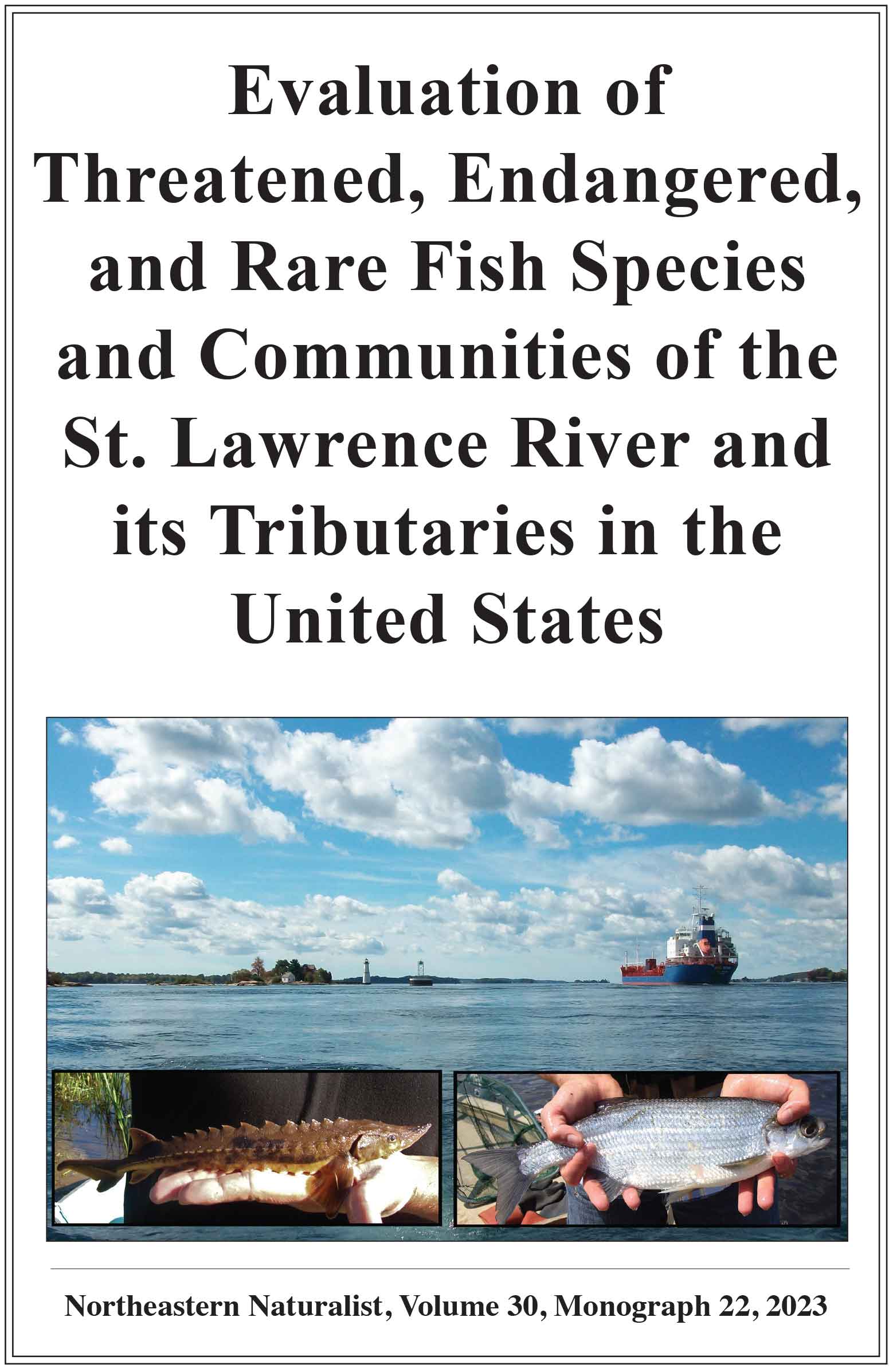
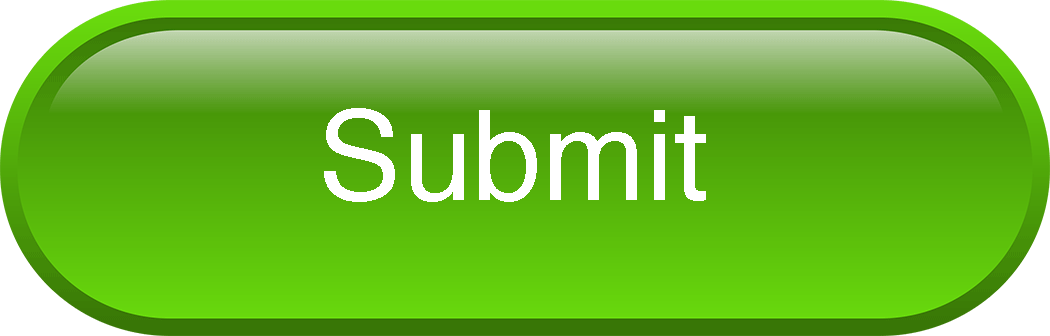
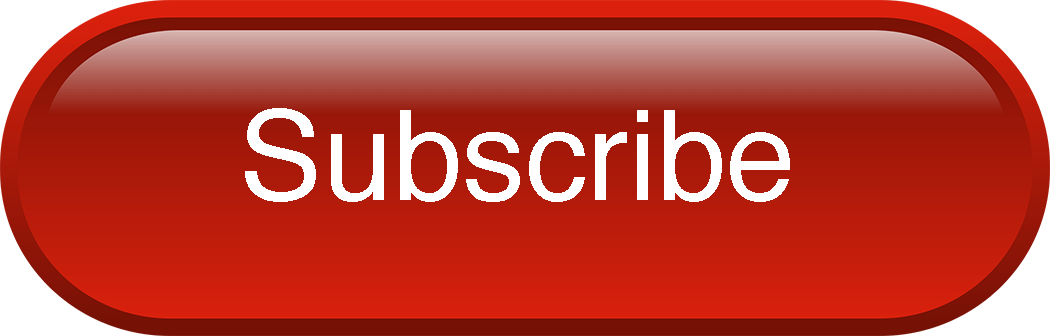
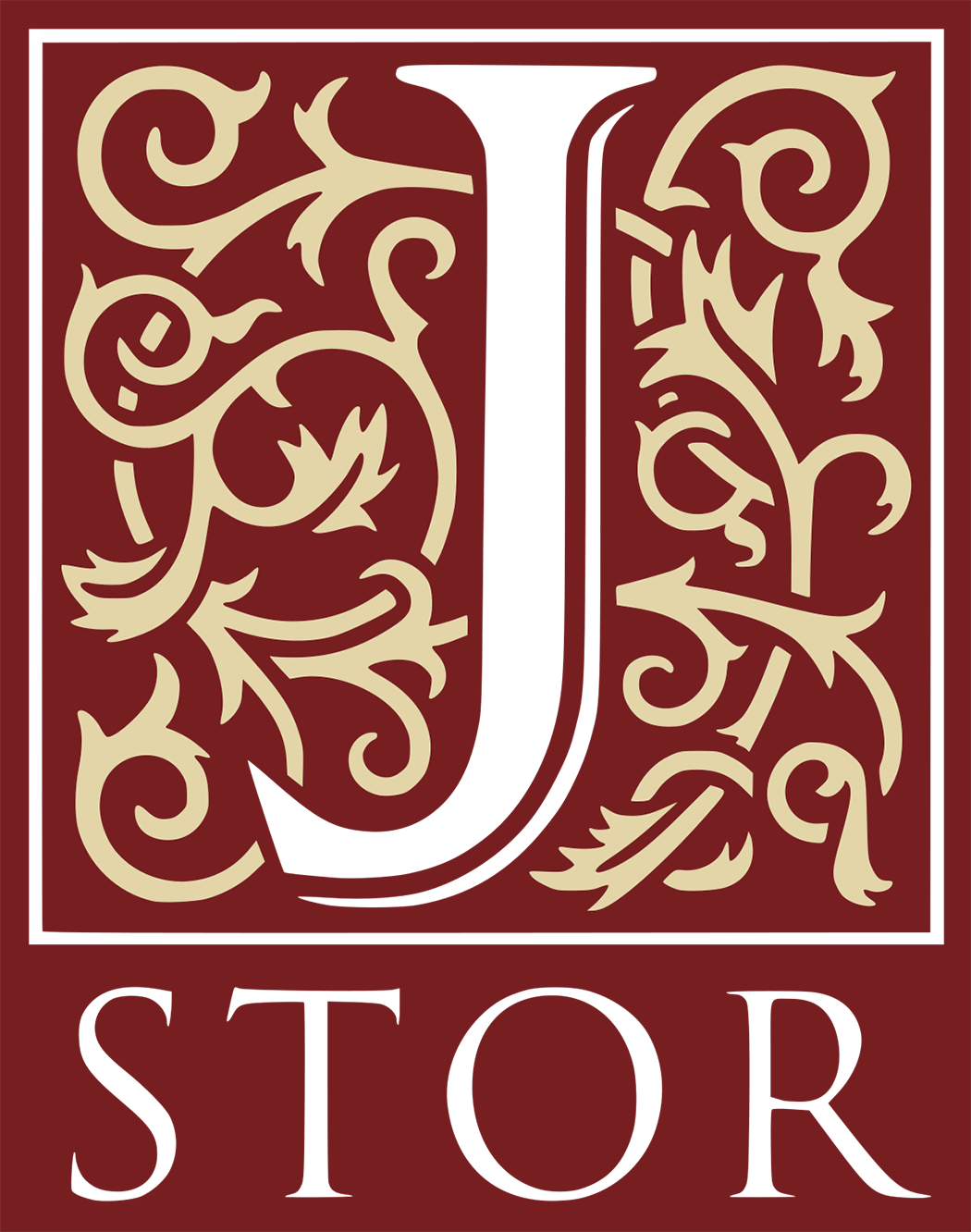
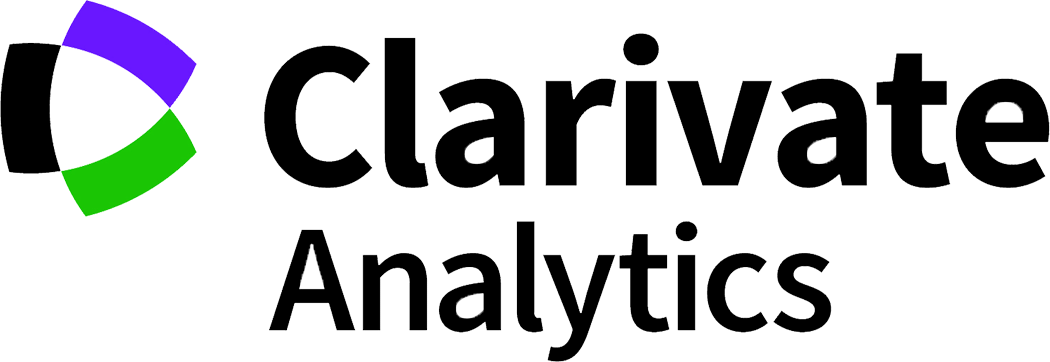
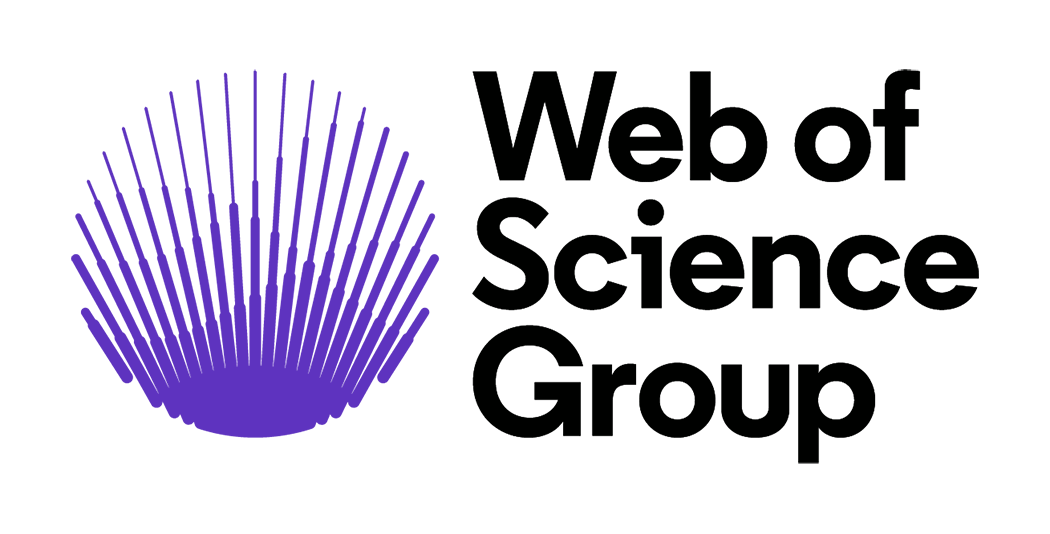
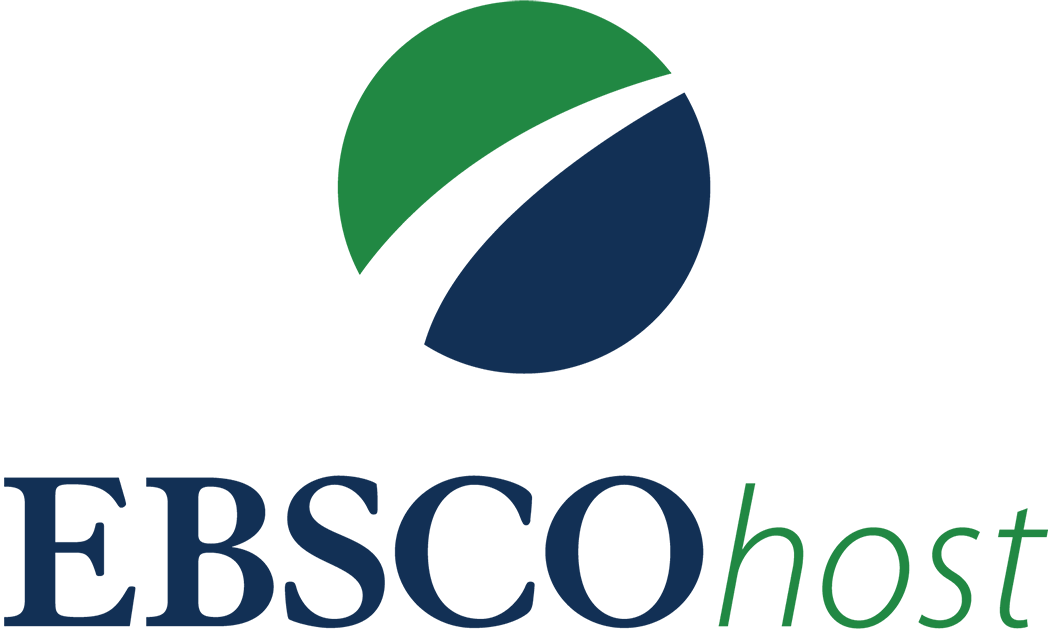
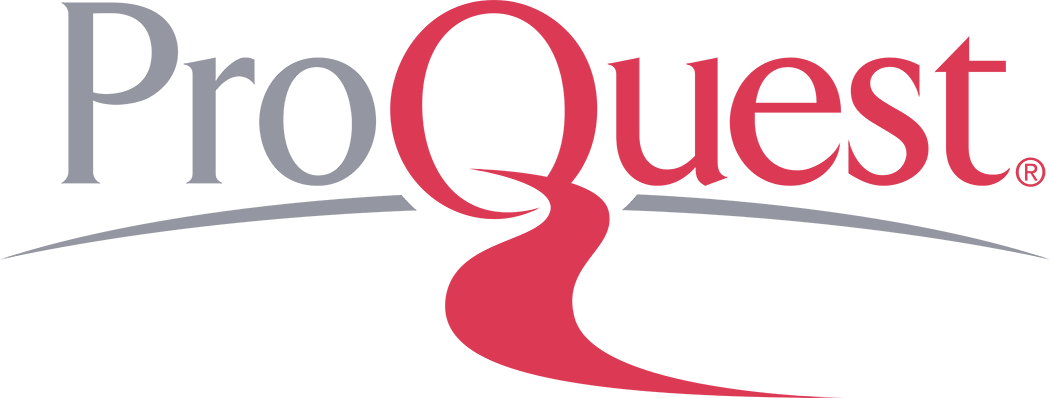
2013 NORTHEASTERN NATURALIST 20(1):103–114
Community Structure and Ecological and Behavioral
Traits of Ants (Hymenoptera: Formicidae) in
Massachusetts Open and Forested Habitats
Israel Del Toro1,2,*, Kevin Towle3, Drew N. Morrison1, and Shannon L. Pelini2,4
Abstract - We investigated ant species richness, interspecific behavioral interactions, and
community composition in adjacent forested and open habitat plots in two forest types of
the northeastern United States: 1) the more common hemlock-White Pine forest studied
at Harvard Forest Long Term Ecological Research Station in central Massachusetts, and
2) the rare Pitch Pine barrens of Myles Standish State Forest in southeastern Massachusetts,
which also provide habitat for multiple rare and endangered species. Overall, we
found that species richness, behavioral interactions, and ecological traits vary between
forested and adjacent open habitat plots. The number of species is five times higher per
plot in the hemlock-White Pine open habitat (compared to forest habitat), but this pattern
(i.e., higher species richness in open vs. forested plots) is not observed in the Pitch
Pine barren site. Non-metric multidimensional scaling analyses suggest that community
composition is significantly different between forest and open plots at both sites. However,
community composition in open plots at both sites did not significantly differ from
each other. We show that behaviorally dominant and submissive species mostly occur in
open plots while neutrally interacting species are more restricted to forested plots, suggesting
that interspecific competitive dynamics may be contributing to the community
assembly patterns observed in open habitats. Our findings suggest that conservation and
management for both open and forested habitat at either site is extremely important when
attempting to maintain optimal ant biodiversity because each habitat type provides suitable
conditions for different suites of ant communities.
Introduction
Communities are structured by various mechanisms including competitive
dynamics, niche partitioning, biotic and abiotic environmental drivers,
and evolutionary pressures on populations (Harrison and Cornell 2008, Parr
and Gibb 2010). Previous studies on the community structure of ants of the
northeastern US have documented species diversity gradients and suggest assembly
rules based on body size across regional scales, but have not provided
assembly rules for local determinants of community composition (Gotelli and
Ellison 2002a, b).
In this comparative and observational study, we investigate species richness
differences (i.e., the number of species per plot) in paired forested and open
habitats at two sites in Massachusetts, the Pinus rigida Mill. (Pitch Pine) barrens
of Myles Standish State Forest (MSSF), and the hemlock-Pinus strobus L.
1University of Massachusetts Amherst, Amherst, MA 01003. 2Harvard University, Petersham,
MA 01366. 3University of Notre Dame, Notre Dame, IN 46556. 4Bowling Green
State University, Bowling Green, OH 43403. *Corresponding author - ideltoro@cns.
umass.edu.
104 Northeastern Naturalist Vol. 20, No. 1
(White Pine) forests at Harvard Forest Long Term Ecological Research Station
(HFLTER). The ants at both sites have been extensively sampled for nearly two
decades, and the overall fauna is relatively well understood (Stefan Cover and
Aaron Ellison, Harvard Forest, Petersham, MA, pers. comm.). The forests of
MSSF are classified as core and critical units of conservation by the Massachusetts
Department of Fish and Game and the Nature Conservancy because they
are habitat for a wide variety of threatened and endangered species of birds,
reptiles, amphibians, and invertebrates (Motzkin et al. 1996, Woolsey et al.
2011).The habitat at HFLTER is more common, but still considered an important
forest habitat core, representative of much of the New England landscape with
significantly lower species diversity (Jenkins et al. 2008, Woolsey et al. 2011).
By understanding fine-scale species richness patterns at these two sites, we aim
to inform management strategies required for the maintenance of ant biodiversity
at sites of conservation interest. Ants are important members of most terrestrial
ecosystems and mediate multiple ecosystem services and processes (e.g., seed
dispersal and nutrient cycling in temperate forests) (Del Toro et al. 2012), and so
conservation of the functional and biological diversity of these animals should be
a research and management priority.
The overarching objective of this study is to compare species richness
and density patterns in forested versus open habitat at two sites (i.e., MSSF
and HFLTER). Specifically this study aims to: 1) compare species richness estimates
at MSSF and HFLTER paired forested and open sites, 2) document natural
history traits that are associated with species occurrence (i.e., behavioral traits
and nesting microhabitat), and 3) compare the community similarity patterns
between MSSF and HFLTER forested and open habitat. We conclude by synthesizing
our findings and offering habitat management suggestions which can be
applied to maximize ant conservation.
Methods
MSSF is located in Plymouth County in eastern Massachusetts (41.8686°N,
70.6688°W) with a mean elevation of 50 m.a.s.l. The habitat is dominated by
Pitch Pine, Quercus ilicifolia Wangenheim (Scrub Oak), and Quercus prinoides
Willdenow (Dwarf Chinquapin Oak) overstory, Vaccinium spp. (blueberries)
in the understory, and extremely sandy soils. HFLTER is located in Worcester
County in central Massachusetts, (42.5391°N, 72.1898°W) with a mean elevation
of 300 m.a.s.l. The habitat is dominated by Tsuga canadensis L. (Eastern
Hemlock) and White Pine overstory, Mitchella repens L. (Partridge Berry) in the
understory, and with soils ranging from sandy to gravelly loams.
We sampled ant nests at ten 5- x 5-m plots at both MSSF and HFLTER (5 forest
habitats and 5 open habitats at each site; n = 20 plots). The forested and open
plots were paired and separated by 200–300 m, and were at least 50 m from any
distinct forest edge, to minimize edge effects on richness patterns. Each pair of
plots was separated from the other pairs by at least 750 m and as much as 10 km;
on average, the distance between the pairs of plots was 2.85 km. We considered
2013 I. Del Toro1, K. Towle, D.N. Morrison, and S.L. Pelini 105
forest plots as those with a closed overstory (tree canopy) and open plots as those
lacking overstory vegetation with an understory dominated primarily by herbaceous
vegetation and grasses. Each 5- x 5-m plot was divided into 1-m2 grids, and
each grid was exhaustively searched for ants (an average of 6 person hours per
plot), which were hand collected and identified to the species level. We recorded
the location of the nest within the grid system and the nesting microhabitat,
which was classified into five categories (Table 1). We also collected ants using
an array of 36 pitfall traps (50-ml Falcon tubes filled with 25 ml of 75% ethanol)
placed at 1-m intervals across the sampling grid and left open to collect ants for
48 hours.
We evaluated interspecific ant interactions at baits using 16 Petri dishes with
2 g of tuna spaced at 3-m intervals in a 9- x 9-m grid (which included the 5-x 5-m
grid where pitfall traps and hand collecting occurred). We observed each dish for
2 minutes, recorded any interspecific interactions, and collected the interacting
ants for species-level identification. We observed each bait station three times
during a 96-minute sampling window (up to 48 possible independent interactions
during the sampling period per plot) and counted the number of aggressive and
neutral interspecific interactions at the baits. The occurrences of ants at baits that
were monopolized by heavy recruitment were only counted once so that each
species occurrence was treated as an independent observation. Interactions were
recorded as dominant, neutral, or submissive. We assigned a classification (i.e.,
dominant, submissive, or neutrally interacting) to species that were observed
interacting a minimum of 5 times based on the majority of their interspecific interactions.
The majority of species that we observed interacting were consistent
in their behavioral response more than 80% of the time. A dominant interaction
consisted of any competitive behavior such as attacks or defenses of resources. A
submissive interaction included retreat, loss of resource allocation, or death resulting
from aggression or stress. A neutral interaction was when neither species
altered their behavior in the presence of the other .
We compared species richness, community composition, nesting microhabitat
type, and number and type of behavioral interactions in open versus
forest habitats at MSSF and HFLTER. We estimated species richness using
rarefaction analyses implemented in EstimateS (Colwell 2009). We completed
the analyses at the site and habitat-type levels (Appendix 1) and used two nonparametric
estimators of species richness for incidence-based data: the ICE
metric and the Chao2 metric, which are appropriate when analyzing species
occurrences (rather than abundances) at pitfall traps (Gotelli et al. 2011). We
present the mean and standard deviation of the observed interspecific interactions
per sampling window (n = 48, unless baits were monopolized by a single
heavily recruiting species; Table 1). We used the Wilcoxon rank sums test to
evaluate if there were differences between forest and open habitat richness
measures, as well as the number and type of behavioral interactions, and nesting
microhabitat type (Table 1).
We used non-metric multidimensional scaling (NMDS) to evaluate community
composition and similarity. We applied the optimal dimensionality of three
106 Northeastern Naturalist Vol. 20, No. 1
Table 1. Wilcoxon rank sums test statistics: Pair-wise comparisons between forest and open plots at Harvard Forest and Myles Standish State Forest.
Total plots = 20; n = 5 per habitat type and forest. We report the mean and standard deviation for observed and estimated species richness as well as the
mean and standard deviation for the counts of observed interspecific interactions (i.e., the number of interactions per observation period at a single bait
station) and the mean and standard deviations of the number of nests and nesting microhabitat types per plot. * indicates significant difference (P < 0.05)
between forest and open plots at a given site.
Harvard Forest Myles Standish
Forested plots Open plots W-stat (P-value) Forested plots Open plots W-stat (P-value)
Diversity measures
Species Observed 3.00 ± 1.00 9.40 ± 4.16 2.50 (0.04)* 8.20 ± 3.11 9.80 ± 2.86 7.00 (0.29)
ICE metric 3.60 ± 2.08 11.91 ± 5.53 2.50 (0.05)* 14.46 ± 5.24 12.96 ± 6.23 15.00 (0.69)
Chao 2 metric 3.19 ± 1.29 10.57 ± 4.69 2.50 (0.05)* 13.45 ± 5.20 11.11 ± 4.23 10.00 (0.69)
Behavioral interactions
Total interactions 1.00 ± 1.25 2.80 ± 2.10 7.50 (0.33) 6.40 ± 4.73 7.20 ± 2.18 10.50 (0.75)
Aggressive interactions 0.20 ± 0.45 2.40 ± 1.10 6.50 (0.09) 0.60 ± 0.89 6.00 ± 2.99 3.50 (0.05)*
Neutral interactions 0.80 ± 0.24 0.40 ± 0.25 16.0 (0.49) 4.00 ± 1.94 1.20 ± 1.31 18.50 (0.19)
Nest density and nesting habitat
Total nests 7.80 ± 2.17 13.40 ± 8.02 5.00 (0.14) 29.80 ± 14.38 32.60 ± 15.37 11.00 (0.77)
Nests in soil 1.40 ± 1.52 11.80 ± 6.98 2.50 (0.04)* 13.60 ± 6.23 23.80 ± 16.23 7.00 (0.29)
Nests in woody debris 0.40 ± 0.89 0.20 ± 0.45 10.00 (0.67) 4.60 ± 3.05 0.80 ± 1.30 22.5 (0.04)*
Nests in vegetation 0.00 ± 0.00 0.00 ± 0.00 NA 0.00 ± 0.00 4.80 ± 4.21 2.50 (0.02)*
Nests in leaf litter 6.00 ± 1.58 0.60 ± 0.89 25.00 (0.01)* 11.80 ± 10.21 3.00 ± 6.71 19.00 (0.18)
Nests under rocks 0.00 ± 0.00 0.80 ± 0.64 5.00 (0.07) 0.00 ± 0.00 0.20 ± 0.42 10.00 (0.42)
2013 I. Del Toro1, K. Towle, D.N. Morrison, and S.L. Pelini 107
axes with a stress value of 0.133. The first two axes of the NMDS explained 96%
of the variation observed in community composition and so are the only ones
reported here. We followed the NMDS with an analysis of similarity (ANOSIM)
to evaluate the overall difference in community composition between forest and
open plots at the two sites. The Wilcoxon rank sums test, NMDS, and ANOSIM
analyses were implemented in R (R-Development-Core-Team 2009) using the
statistical package “vegan” (Oksanen et al. 2010).
Results and Discussion
Observed and estimated species richness was five times higher at HFLTER
in open compared to forested plots. Species richness did not significantly differ
between MSSF open and forest plots (Table 1, Appendix 1). Additionally, species
richness at HFLTER open plots did not significantly differ from either forest
or open plots at MSSF. The higher species richness at the HFLTER open plots
conforms to a global pattern described for ants in open and warmer habitat types
(Andersen 1995, 1997). We hypothesize that the open nature of the environment
allows ants to be more active and less thermally constrained, as opposed to
forested sites, where cool and damp conditions may be acting as a habitat filter
(Lessard et al. 2009). At MSSF, the numbers of species did not differ significantly
between forest and open sites but remained high (compared to HFLTER) in both
forest and open plots. This finding could be an indication that additional environmental
factors (e.g., the sandy soil type) might be contributing to the observed
high ant abundances, which may ultimately lead to higher species richness.
Another factor that can potentially explain the high richness patterns observed
at MSSF is the open nature of the canopy in the Pitch Pine barren habitat, which
increases habitat complexity in the understory and makes more nesting niches
available, a pattern suggested for Massachusetts forests (Woolsey et al. 2011).
We observed behavioral interactions for 15 species (Appendix 2) based on
169 observed interactions at the bait stations. Based on our observations, we
were able to classify 13 of the 15 species as either “dominant”, “submissive”, or
“neutral” interacting species. The number of aggressive and neutral interactions
at HFLTER did not differ between forest and open habitat. However, a larger
sample size may increase support for the non-significant trend (P = 0.09), which
may suggest that more aggressive interactions may be occurring in open habitat
at HFLTER. In MSSF, there were significantly more aggressive interactions in
open plots than in forest plots (P = 0.05; Table 1), despite there being no differences
in the total number of interactions between open and forested plots.
The number of observed interspecific interactions only considers a subset of the
regional species pool; however, this observational study may be more reflective
of the realistic interactions between ground-foraging ant species of the region, in
particular when they are competing for a limited, high-quality food resource.
We found that within-site nest densities between forested and open plots did
not significantly differ. However, while there were significantly fewer nests in
the forested plots at HFLTER than in MSSF (P = 0.011), there was no significant
108 Northeastern Naturalist Vol. 20, No. 1
difference in nest densities between HFLTER and MSSF open plots (P = 0.121).
The patterns of densities may partially explain why richness was significantly
lower in HFLTER forest plots and than all other plots. We hypothesize that in
Massachusetts forests, there exists a positive correlation between species richness
patterns and local species densities. Furthermore, at HFLTER, we found
significantly more nests in soil in open plots than in forest plots, and more nests
in leaf litter in forest plots than in open plots (differences in these nesting microhabitats
at MSSF were not significant). At MSSF, we found more nests in woody
debris in forest plots than in open plots, and found more nests under rocks in
open habitat than in forested habitat. These findings highlight the microhabitats
in which ant nests were mostly collected and may prove to be important determinants
of nesting preferences that should be further examined in subsequent
studies and may provide important details about conservation and management
of ants for these forests.
Nonmetric multidimensional scaling analyses show that local communities
in forest and open habitat at MSSF and HFLTER are significantly different from
each other. Species composition in the open sites of MSSF and HFLTER were not
different from each other as indicated by the overlapping 95% confidence intervals
(Fig. 1). In contrast, we found that forested-plot ant communities at HFLTER
and MSSF are significantly different from each other, but still more similar to
each other than to open-plot communities at either HFLTER or MSSF (Fig. 1).
The ANOSIM results (R = 0.563, P = 0.001) suggest that a significant amount of
variation in ant community composition is predictable based on habitat type (i.e.,
forest vs. open).
For the species that were observed interacting at the baits, neutrally interacting
species were mainly found in forested plots, while aggressive/submissive
interacting species were mainly found in open habitat plots (Fig. 1). The neutrally
interacting species that were commonly collected in forested plots included
Camponotus noveboracensis Fitch (New York Carpenter Ant), C. pennsylvanicus
DeGeer (Eastern Carpenter Ant), Lasius alienus Foerster (Cornfield Ant),
Aphaenogaster picea Wheeler (Pitch Black Ant), Myrmica punctiventris Roger
(Punctured Ant), and Temnothorax curvispinosus Mayr (Bent-spined Ant). This
suite of species was exclusively collected in forested plots and was not observed
in open habitat. The aggressive species that dominated open habitat were Lasius
neoniger Emery (Labor Day Ant), Crematogaster lineolata Say (Small-lined
Ant), and Monomorium emarginatum DuBois (Furrowed Ant), and the submissive
species that were commonly collected in open habitat were Dolichoderus
pustulatus Mayr (Common Bog Ant), Tapinoma sessile Say (Odorous House
Ant), Formica incerta Buren (Uncertain Ant), and F. pallidefulva Latreille
(Pale Ant). These species were more commonly associated with open habitat or
edge habitat, but F. pallidefulva was occasionally collected in some of our forest
pitfall traps (a total of 9 individuals from 2 pitfall traps).
Based on the observed interactions, we hypothesize that competition for food
resources is also likely to contribute to the structure of communities in open habitat
plots, but this is a question that remains to be tested in future studies. It is also
2013 I. Del Toro1, K. Towle, D.N. Morrison, and S.L. Pelini 109
likely that in northern latitudes, environmental variables such as temperature can
interfere with how a species behaves, such that a species may be less thermally
constrained and subsequently more behaviorally aggressive in open habitats. We
observed this to be the case with edge species like Formica subsericea Say (Silky
Ant), which was predominantly neutrally interacting in forested plots but could
occasionally be aggressive in open plots. Behavioral shifts have been noted to be
context dependent and should be further experimentally studied in species like
F. subsericea, which commonly occurs in both habitat types, to identify the exact
mechanisms that cause behavioral shifts.
The data compiled here suggest that both forested and open habitat is necessary
for maintaining ant biodiversity. The forests of HFLTER and MSSF
are unique in their species composition (Fig. 1), but those of MSSF support
far more species (Appendices 1, 2). Open habitat is particularly important in
Figure 1. Nonmetric multidimensional scaling plot. Depicts plots as solid symbols
(solid circles = HFLTER forested; squares = MSSF forested; stars = HFLTER open; solid
triangles = MSSF open). Ellipses show 95% confidence intervals around plot symbols.
Additional symbols are species scores classified based on observed behavioral interactions
(hollow circles = submissive species; hollow triangles = dominant species; crosses
= neutral species).
110 Northeastern Naturalist Vol. 20, No. 1
HFLTER since this habitat supports up to five times the number of species,
including ecologically important species (e.g., Aphaenogaster picea, Formica
spp.), than forested habitat (Appendix 1). Open habitat in MSSF is also important
because the community structure (not species richness) is different in forest
and open plots (Fig. 1), and therefore, maximizing biodiversity conservation
requires the appropriate management for both habitat types. It is also important
to note that the pine barren habitat of MSSF is rare in the northeastern US and
is home to many species of conservation concern (Motzkin and Foster 2002,
Motzkin et al. 1996, Woolsey et al. 2011). As an example, MSSF is one of few
sites in the northeastern US where rare ant species like Formica knighti Buren
(Knight’s Ant), Monomorium viridae Brown (Green Monomorium), and a
new species of socially parasitic Nylanderia have been collected (Ellison et al.
2012). While the forest type at HFLTER is more common in the New England
landscape, conservation efforts should consider that the maintenance of early
successional open habitat is necessary for optimally managing ant biodiversity,
which has been a topic considered in the conservation efforts of bird species
(DeGraaf and Yamasaki 2003) but never considered from the perspective of invertebrate
conservation for the region.
Acknowledgments
The authors would like to thank the Harvard Forest LTER Research Experience for
Undergraduates program for supporting this work (through NSF grant DBI 10-03938)
and a National Science Foundation Graduate Fellowship award to I. Del Toro. We thank
Michael Marquis and Natashia Manyak for their help in the field and processing samples.
Additionally we thank Nathan Sanders, Relena Ribbons, Aaron Ellison, and two anonymous
reviewers for their comments on earlier drafts of this short communication.
Literature Cited
Andersen, A.N. 1995. A classification of Australian ant communities, based on functional
groups which parallel plant life-forms in relation to stress and disturbance. Journal of
Biogeography 22:15–29.
Andersen, A.N. 1997. Functional groups and patterns of organization in North American
ant communities: A comparison with Australia. Journal of Biogeography 24:433–460.
Colwell, R.K. 2009. EstimateS: Statistical estimation of species richness and shared
species from samples. Available online at http://purl.oclc.org/estimates. Accessed 1
March 2012.
DeGraaf, R.M., and M.Yamasaki. 2003. Options for managing early successional forest
and shrubland bird habitats in the northeastern United States. Forest Ecology and
Management 185:179–191.
Del Toro, I., R.R. Ribbons, and S.L. Pelini. 2012. The little things that run the world re -
visited: A review of ant-mediated ecosystem services and disservices (Hymenoptera:
Formicidae). Myrmecological News 17:133–146.
Ellison, A.M., N.J. Gotelli, E.J. Farnsworth, and G.D. Alpert. 2012. A Field Guide to the
Ants of New England. Yale University Press, New Haven, CT. 350 pp.
Gotelli, N.J., and A.M. Ellison. 2002a. Assembly rules for New England ant assemblages.
Oikos 99:591–599.
2013 I. Del Toro1, K. Towle, D.N. Morrison, and S.L. Pelini 111
Gotelli, N.J., and A.M. Ellison. 2002b. Biogeography at a regional scale: Determinants of
ant species density in New England bogs and forests. Ecology 83 :1604–1609.
Gotelli, N.J., A.M. Ellison, R.R. Dunn, and N.J. Sanders. 2011. Counting ants (Hymenoptera:
Formicidae): Biodiversity sampling and statistical analysis for myrmecologists.
Myrmecological News 15:13–19.
Harrison, S., and H. Cornell. 2008. Toward a better understanding of the regional causes
of local community richness. Ecology Letters 11:969–979.
Jenkins, J.C., G. Motzkin, and K. Ward. 2008. The Harvard Forest flora. An inventory,
analysis, and ecological history. Harvard Forest 28:1–266.
Lessard, J.P., R.R. Dunn, and N.J. Sanders. 2009. Temperature-mediated coexistence in
temperate forest ant communities. Insectes Sociaux 56:149–156.
Motzkin, G., and D.R. Foster. 2002. Grasslands, heathlands, and shrublands in coastal
New England: Historical interpretations and approaches to conservation. Journal of
Biogeography 29:1569–1590.
Motzkin, G., D.R. Foster, A. Allen, J. Harrod, and R. Boone. 1996. Controlling site to
evaluate history: Vegetation patterns of a New England sand plain. Ecological Monographs
66:345–365.
Oksanen J., R. Kindt, P. Legendre, R.B. O’Hara, G.L. Simpson, P. Solymos, H.H. Stevens,
and H. Wagner. 2010. Vegan: community ecology package.in R. p. v. 1.17-4.
Available online at http://cc.oulu.fi/~jarioksa/softhelp/vegan.html. Accessed 1
March 2012.
Parr, C.L., and H. Gibb. 2010. Competition and the role of dominant ants. Pp. 77–96,
In L. Lach, C.L. Parr, and K.L. Abbot (Eds.). Ant Ecology. Oxford University Press,
Oxford, UK. 401 pp.
R-Development-Core-Team. 2009. R: A language and environment for statistical computing.
Vienna, Austria. Available online at http://www.r-project.org/. Accessed 1
March 2012.
Woolsey, H., A. Finton, and J. DeNormandie. 2011. BioMap 2: Conserving the biodiversity
of Massachusetts in a changing world. Massachusetts Department of Fish and
Game and The Nature Conservancy, Boston, MA.
112 Northeastern Naturalist Vol. 20, No. 1
.Appendix 1. Rarefaction and species richness estimation (a), number of species observed
(b), and Number of species estimated (c), derived from incidenc e-based estimators.
2013 I. Del Toro1, K. Towle, D.N. Morrison, and S.L. Pelini 113
Appendix 2. Species list for HFLTER and MSSF with natural history observations. Sites: MS = Miles Standish State Forest, HF = Harvard Forest LTER, 2011
= sampled in 2011, and PC = previous collections. Behavioral interactions: D = dominant, S = submissive, N = neutral, and N/A = not applicable. Nesting
habitat: S = soil, WD = woody debris, LL = leaf litter, V = vegetation, and UR = under rocks. Colony size: A = 10 –100 individuals, B = 101–1000 individuals,
C = 1001–10,000 individuals, and D = >10,000 individuals.
Sites Behavioral interactions Nesting habitat Colony size
Subfamily Genus Species MS HF 2011 PC D S N N/A S WD LL V UR A B C D
Amblyoponinae Amblyopone pallipes • • • • •
Dolichoderinae Dolichoderus mariae • • • • • • • • •
Dolichoderinae Dolichoderus pustulatus • • • • • •
Dolichoderinae Tapinoma sessile • • • • • • • • • •
Formicinae Brachymyrmex depilis • • • • •
Formicinae Camponotus americanus • • • • • •
Formicinae Camponotus noveboracensis • • • • • • • •
Formicinae Camponotus pennsylvanicus • • • • • • •
Formicinae Formica dolosa • • • •
Formicinae Formica incerta • • • • • • •
Formicinae Formica knighti • • • • •
Formicinae Formica lasiodes • • • • • • • • • •
Formicinae Formica pallidefulva • • • • • • •
Formicinae Formica neogagates • • • • • • •
Formicinae Formica rubicunda • • • • •
Formicinae Formica subsericea • • • • • • • • • •
Formicinae Lasius alienus • • • • • • • • • •
Formicinae Lasius claviger • • • • • • • • •
Formicinae Lasius flavus • • • • • • •
Formicinae Lasius nearcticus • • • • • • •
Formicinae Lasius neoniger • • • • • • • • • • •
Formicinae Lasius umbratus • • • • • • •
Formicinae Nylanderia parvula • • • • • • • • • •
Formicinae Nylanderia sp. • • • • •
Formicinae Prenolepis imparis • • • • • • • •
Mymicinae Aphaenogaster picea • • • • • • •
114 Northeastern Naturalist Vol. 20, No. 1
Sites Behavioral interactions Nesting habitat Colony size
Subfamily Genus Species MS HF 2011 PC D S N N/A S WD LL V UR A B C D
Mymicinae Aphaenogaster rudis • • • • • • • • • • •
Mymicinae Crematogaster cerasi • • • • • • •
Mymicinae Crematogaster lineolata • • • • • • • • • •
Mymicinae Monomorium emarginatum • • • • • • • •
Mymicinae Monomorium viridae • • • • •
Mymicinae Myrmica americana • • • • • • • • • •
Mymicinae Myrmica detrinodis • • • • •
Mymicinae Myrmica puntiventris • • • • • • • • • • •
Mymicinae Myrmica scuptilis • • • • • • •
Mymicinae Myrmica smithana • • • • • • •
Mymicinae Myrmecina americana • • • • • • •
Mymicinae Pheidole pilifera • • • • • • • • •
Mymicinae Solenopsis molesta • • • • • • • •
Mymicinae Stenamma brevicorne • • • • • • •
Mymicinae Stenamma schmitti • • • • •
Mymicinae Temnothorax curvispinosus • • • • • • • • • • •
Mymicinae Temnothorax longispinosus • • • • • • •
Mymicinae Temnothorax schaumii • • • • • •
Mymicinae Tetramorium caespitum • • • • • •
Ponerinae Ponera pennsylvanica • • • • • • • •